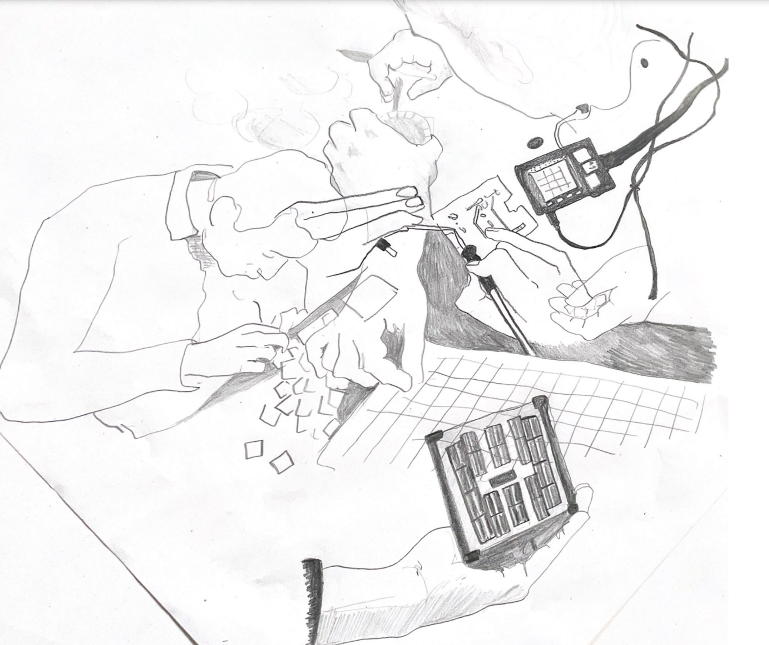
A stack of breadboards sitting around. The end pieces of solder are on the floor. Instruments for measuring, connecting, and separating physical, electrical, and chemical components. These are the materials of the Maker, DIY, and open hardware movements. They are the tools of the future that can lead to a better return on investment, increase the accessibility of science and put the power of monitoring climate change into the hands of communities. They allow for critical making to occur, with impactful learning throughout the process of creation, use, and troubleshooting.
And yet, the climate crisis is here. Coal mining and processes of oil and gas exploration and extraction are actively being called on to be reduced. Yet, the exploitative human labor practices used to mine the rare metals necessary for our everyday gadgets, vehicles, and tools of science, receive less attention. As makers, doers, practitioners and researchers engaged in the use of seemingly reusable materials, but not necessarily replenishable, we have not done a sufficient job in interrogating the relationship between the seemingly sustainable tools we use and their role in the further exacerbation of human exploitation and local environmental loss.
To begin exploring supply chains and the environmental impact of open technology, with several others, we’re designing a workshop that will dive into questions around broad impact. We hope to develop a modular resource for people who want to assess the sustainability of individual tools, in order to minimize the environmental impact of open hardware projects in the future. We plan to do this by bringing together tool developers, users, sustainability experts, and policymakers to identify major sustainability concerns, understand how to open frameworks uniquely influence environmental impact and analyze previous initiatives that have enabled sustainable and circular products.
One of the ways to work through these questions is through a product lifecycle approach. The product lifecycle is broken down into stages such as raw material extraction, manufacturing, use, disposal, and transportation. For each stage of the lifecycle, the associated material, energy, and water consumed as well as the greenhouse gas emitted from a given product can be evaluated to understand the overall environmental impact of the product and point to areas of improvement.
Let’s consider an open-source air quality sensor. The raw material extraction stage would consider all the components that make up the sensor—the breadboard, microcontroller, pollutant sensor, and LEDs—as well as the metals, plastics, and other materials that make up each component. At the manufacturing stage, we look at the processes and materials used to bring the individual components together to create the tool—the soldering, gluing, and even physical labor requirements. In these stages, we ask questions such as: where do these materials come from? How were they extracted? What are the inputs needed to manipulate them into their functional state?
The use stage would look at the inputs required for the sensors to be used, such as charging a battery or connection to wifi, how frequently it is used, and how many times it can be used before reaching its end of life. The disposal stage takes into account all the end-of-life processes associated with the tool, such as whether or not parts of the tool can be recycled or reused and how parts are disposed of. The transportation stage occurs between and during the other stages, looking at how raw materials and their waste materials are transported, how far and the air quality sensor itself during the extraction, manufacturing, use, and disposal stages.
Beyond the air quality sensor itself, there are additional lifecycles involved in its journey to advance environmental causes. To calibrate sensors, multiple sensors may be needed to record data simultaneously. For the data collected by the sensor to be recorded, some sort of communication system is needed to transfer the data to a computer. Within the computer itself, the air quality data will need to be processed, analyzed, and shared. Depending on the efficiency of software and programs used, making air quality data “usable” can require large amounts of energy and thus GHG emissions. For instance, the training phase of natural language processing models used in artificial intelligence of environmental applications, such as air quality predictions and monitoring climate change misinformation, produces GHG emissions equivalent to 300 flights between New York and San Francisco. This is due to the large computation time and resources. The computer, software, communication systems, and devices all have their own individual lifecycles with varying environmental impacts that are tied to the use of an air quality sensor.
We are also interested in understanding how “openness” itself impacts the lifecycle of open hardware. For example, unlike proprietary hardware, with access to tools and materials, anyone can use public blueprints to build their own tool. Open hardware is often lower cost, which further expands access to more and new tool users. On one hand, this accessibility could mean more tools—more extraction of materials, more energy used, and more waste. Low cost can be associated with shorter tool lifetimes as well. On the other hand, open hardware can be made locally or on demand, shifting manufacturing from mass production to on-demand. This can both minimize transportation impacts and the overproduction of goods.
So while we actively encourage the next generation of tools and devices that will help us to measure, monitor, understand and address environmental loss and climate change, we should also be mindful of the impact of those tools. Using sustainability assessment models can help developers, researchers and home practitioners understand the materials they use, and where they can reduce environmental impact. We hope that through our upcoming work we can offer a model that helps people tackle the intricate connectivity between tools, their building blocks, and the environment. Designing and using tools in a way that minimizes environmental impact will ultimately maximize these tools’ efforts in accelerating environmental research and in empowering the communities that use them.